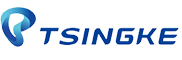
Expression System |
Introduction
|
Advantages
|
Escherichia coli |
E. coli stands out as a widely recognized expression system, readily amenable to genetic manipulation, facilitating cost-effective and high-yield production of various recombinant proteins, including cytokines, enzymes, immunogens, and antibody fragments.
|
- Cost-effective;
- Fast;
- Flexible to scale up;
- Simple transformation operations;
- Protein expression can be optimized through multiple parameters.
|
Pichia pastoris |
The Pichia expression system is highly successful for foreign protein expression, avoiding endotoxin problems seen in bacteria and viral contamination in animal cell cultures. Pichia pastoris, in particular, enables essential posttranslational modifications typical of higher eukaryotes, including signal sequence processing, disulfide bridge formation, and glycosylation.
|
- High expression levels: Utilizing the methanol-inducible alcohol oxidase I (AOX1) promoter enables precise control of the expression of exogenous genes; cost-effective;
- Rapid growth with simple cultivation conditions;
- Various host strains and expression vectors are available for intracellular or secretory expression;
- Efficient protein secretion allows for easy purification and post-translational protein processing, ensuring correct folding and modification of the exogenous protein; superior N-terminal glycosylation capability compared to brewing yeast;
- Low endotoxin levels.
|
Baculovirus |
Insect cells are a versatile host for expressing a variety of recombinant proteins. Their strong folding capacity and high culture density make them ideal for expressing complex intracellular and viral proteins. Notably, the HPV vaccine Cervarix, produced in insect cells as virus-like particles (VLPs), was approved for human use in 2007. Beyond therapeutic and vaccine applications, insect cell-produced highly active proteins are widely employed in disciplines like biophysics and biochemistry for structural analysis, drug design, and the development of diagnostic reagents.
|
- High expression levels, especially for complex intracellular and viral proteins; Rapid growth rate;
- Strong folding capability for complex proteins;
- Moderate scalability;
- Extensive post-translational modifications; Glycosylation similar to mammalian cells; The relative ease of enzymatic deglycosylation is advantageous for protein structure research.
- Capable of producing virus-like particles (VLP), such as for HPV vaccine production;
- Low endotoxin levels.
|
Mammalian |
Mammalian cells have become the preferred host cells for the manufacture of a wide range of biopharmaceuticals. Recombination proteins expressed by the mammalian cell expression system approximate human forms due to post-translation modification, and the mammalian cell expression system is used widely to manufacture therapeutic recombinant proteins.
|
- High expression levels;
- Moderate scalability;
- The suspension culture characteristics of cells allow for large-scale production;
- Effective protein folding;
- Suitable for protein secretion;
- Ample post-translational modifications;
- Low endotoxin levels.
|
Bacillus subtilis |
Bacillus subtilis, as a Gram-positive bacterium, is considered an ideal host for expressing and secreting foreign proteins in prokaryotic expression systems. Its characteristics of non-pathogenicity, strong protein secretion capabilities, and well-established fermentation foundation and production technologies contribute to its significance as a crucial model strain in prokaryotic expression systems.
|
- Non-pathogenic;
- Simple cell wall composition;
- Low codon bias;
- High solubility of expressed proteins;
- Suitable for efficient secretion expression of heterologous proteins;
- Good biological activity.
- Low endotoxin levels;
|